Richard P. Shefferson, University of Tokyo, discusses his article: ‘Life history costs drive the evolution of mycoheterotrophs: increased sprouting and flowering in a strongly mycoheterotrophic Pyrola species’
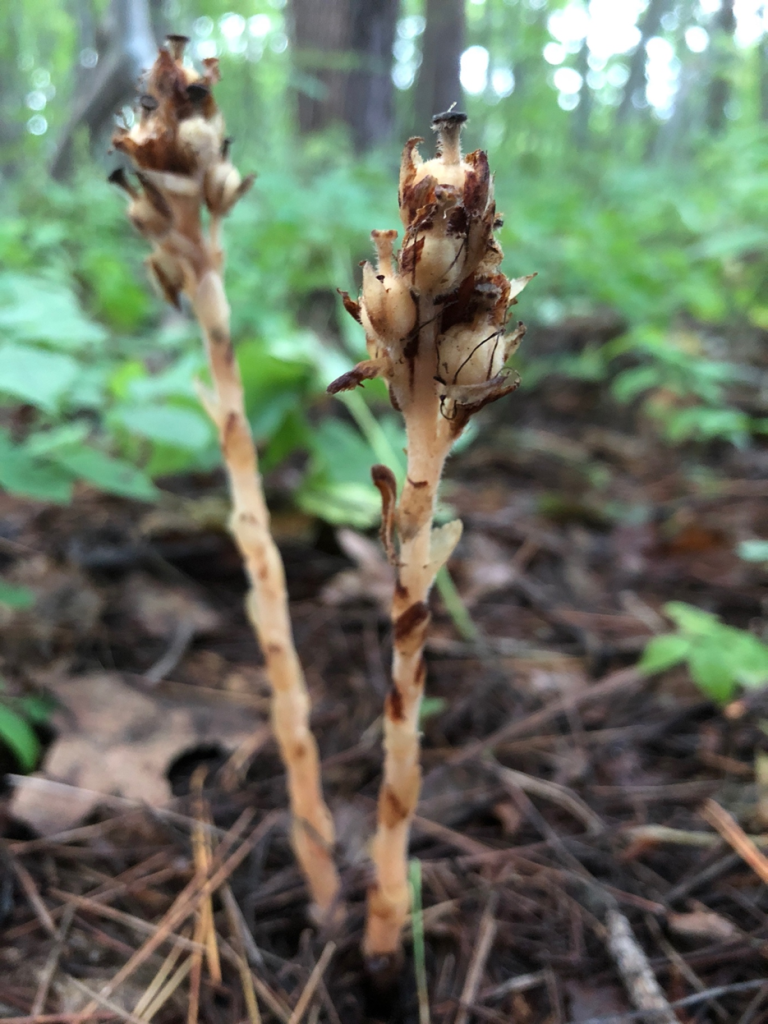
Background
When we think of plants, we think of trees and grasses, flowers, and ferns. The single most obvious and important characteristic of plants is that they are green, and that alludes to their uniquely important role in producing the dioxygen that we live on while fixing carbon for their own use. And yet, the plant kingdom also includes many examples of species that do not photosynthesize, or at least do not rely exclusively on photosynthesis to provide them with chemical energy. Indeed, it is difficult to ignore that some have diversified into large clades of non-photosynthetic species, as in the case of the monotropes (Figure 1).
Many plant species are non-photosynthetic. These plants come in a variety of forms, but as far as we know, all have evolved from relying on photosynthesis for their carbon needs, to relying on their mycorrhizal fungi. Procuring carbon from mycorrhizal fungi in addition to the typical mineral nutrients that mycorrhizae provide is a phenomenon known as mycoheterotrophy. In the process of becoming mycoheterotrophs, these plants have generally become parasites since mycorrhizal fungi are heterotrophic, so any carbon loss that these fungi experience should be harmful to their survival or reproduction.
Our study system
But how did these plants become non-photosynthetic? To find out, my co-authors and I embarked on a long-term demographic study of two sister species of the genus Pyrola, P. japonica and P. subaphylla. Although this genus is green, it is odd in that its species often exhibit strong evidence of partial mycoheterotrophy. Both species produce green leaves, so the carbon that they take in is a mix of photosynthetically fixed carbon and carbon from their mycorrhizal fungi, the latter of which is ultimately derived from some nearby ectomycorrhizal trees. However, P. subaphylla’s carbon and nitrogen isotope ratios suggest that it is almost exclusively mycoheterotrophic, while P. japonica’s isotope ratios suggest that it is less so. The plants are small-statured perennial herbs that grow on the floors of Japanese mid-elevation forests (Figures 2 and 3). Both produce small leaves, but P. subaphylla produces many fewer leaves, all of which are a good deal smaller than those of P. japonica.

Our findings
We tracked the demography of nearby populations of these two species every year from 2015 to 2020, near the iconic volcano Mt. Bandai in western Fukushima Prefecture, Japan (Figure 4). This sort of field work is one of the joys of working on the demography of herbaceous plants, and students enjoyed participating every year. We found that, relative to its less mycoheterotrophic cousin, P. subaphylla exhibited strong life history costs, most notably a strong cost of growth to survival and costs of reproduction to sprouting, size, and future reproduction. And yet, even with these costs, it was four times more likely to flower, and significantly more likely to sprout, though to smaller sizes. Our evidence also suggests that underground seedlings survived and matured at roughly twice the rate of P. japonica. Interestingly, increases in flowering led to increases in fitness, even though P. subaphylla was more likely to die after flowering, while shifts in transitions away from sprouting led to decreased fitness. All in all, this paper shows what we believe is the first evidence of costs of growth and reproduction driving the evolution of increased mycoheterotrophy.

Interpretation
Why do these patterns occur? The evidence would suggest that P. subaphylla is better at exploiting its mycorrhizal fungi for carbon than P. japonica. In line with the grand écart hypothesis, partial mycoheterotrophy appears costly but likely represents an important means of supplementing and stabilizing the demography of the individual. This further suggests that this clade may be en route to evolving full mycoheterotrophy, complete with the loss of green leaves. So, grab a fermented beverage, and enjoy the product of a human-fungal symbiosis while you wait a few millennia to find out if this happens. This new paper is also meant to provide a workable example showcasing the R package lefko3 as a powerful tool to solve questions in ecology and evolution. The analysis included the construction of function-based historical matrix models, which are essentially like discretized complex integral projection models (IPMs) except with states at three consecutive times represented by each matrix, and stochastic life table response experiment (SLTRE) analysis. The full demographic dataset used in the paper is now included as a part of lefko3, and the new paper includes a supplementary R Notebook file that reproduces the entire analysis. We urge readers interested in learning to program with this package to explore that supplement.